گوجه فرنگی به عنوان باتری ساعت
گوجه فرنگی به عنوان باتری ساعت
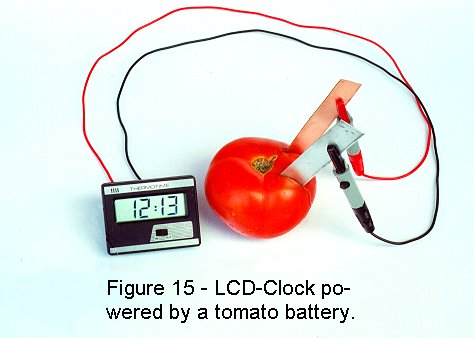
آهن یون مس را از محلول الکترولیت آن جدا می کند
In 1791 Luigi Galvani discovered electrical activity in the nerves of the frogs that he was dissecting. He thought that electricity was of animal origin and could be found only in living tissues. A few years later, in 1800 Alessandro Volta discovered that electricity could be produced through inorganic means. In fact, by using small sheets of copper and zinc and cloth spacers soaked in an acid solution, he built a battery – the first apparatus capable of producing electricity. Naysayers were quick to predict that electricity would never serve a useful purpose. Obviously they were very wrong. Electricity has a central role in our lives and to this day Electrochemistry is a standard course of study.
While listening to lessons on Electrochemistry, many students may wonder why it was ever invented, if it was really ever necessary to invent it and if the world would be better off without it. With the small experiments that follow, we hope to make peace between these students and the study of Electrochemistry. These fun and simple experiments can teach the fundamental concepts of Electrochemistry without asking much of the student. As you will see, many of these demonstrations are easily adapted to various configurations and each can be done independently or as part of the full curriculum.
POROUS VASE – An actual porous vase made for the purpose may be difficult to acquire. It is used to prevent the quick mixing of various solutions, while permitting the exchange of ions. For our purposes you can adapt a terracotta pot of the type used in gardening simply by plugging the hole in the bottom with molten wax and allowing it to cool. Another even more economical answer lies in constructing a barrier of paper. As shown in figure 4, roll the paper to form a cylinder and glue it in place on the bottom of the main container using a silicone adhesive such that liquids cannot pass between the two areas defined by the paper. A barrier of just one sheet would be too permeable, therefore use at least three layers of paper when building this device.
DISTILLED WATER – Don’t use de-ionized water in place of actual distilled water. Many times water sold for use in household irons is de-ionized rather than distilled water. Pharmacies sell actual distilled water. What is the difference? Many substances are soluble in water and a few of these substances separate into positive and negative ions in water. Generally these are made up of molecules that have ionic bonds, while non-ionic molecules remain intact, just in solution. For example, sugar dissolves easily in water and the sugar molecules remain intact as sugar. De-ionized water can have any number of dissolved substances that do not result in ions, yet are present nonetheless. Apart from this, a poor quality sample of de-ionized water can contain significant amounts of ions. On the other hand, distilled water is usually very pure – containing only actual water molecules.
WHERE TO OBTAIN THE MATERIALS – You can buy the chemicals in a store that sells chemicals and laboratory equipment. Often, this type of store is located near universities. Some grocery or hardware store are able to get you the chemicals needed for these experiments. You can often purchase copper and zinc strips at a hobby retailer or hardware store. You can also ask a firm which works with sheets of these metals for some pieces, for example a gutter-pipe firm and a zinc galvanic treatments firm. For the first trials, you can also use a piece of copper and a zinc-galvanized nail or screw.
CATHODE AND ANODE – In the course of these experiments, often I’ll speak of cathodes and anodes. As you may notice, in some cases the cathode has been assigned a positive polarity and, in other cases, a negative one. This is due to the cathode being the electrode where the reaction of reduction occurs. In the case of a battery, this reduction occurs spontaneously and consumes electrons, so the cathode garners a positive charge. In the case of an electrolytic cell, the reduction is forced, producing electrons, so the cathode carries a negative charge. The anode will have in each case the polarity opposite the cathode’s.
SOLUTIONS (IMPORTANT) – The dilution of acids is dangerous. If water is added to a concentrated acid, it can explode violently causing severe injuries. Never pour water into concentrated acid. Always add the acid to the water. If you need to dilute an acid, get help from a Chemist. When making a solution of copper sulfate or zinc sulfate, add these chemicals to water rather than adding the water to the chemical.
OTHER PRECAUTIONS – Do not allow any of the chemicals discussed in these experiments to get on your hands or skin. Do not put them in your mouth or eat them. Do not breathe any vapors from these chemicals. Do not keep them in bottles or containers that could be confused with a food or drink container. Do not leave these chemicals in such a place that it might be confused with a food or drink (such as on a kitchen table or counter or in the refrigerator). Store them in a separate, controlled place away from foods and out of the reach of children. Label each container clearly with the name of the contents and as a non-food item.
|
Materials: – a battery Using the various items listed above, construct the device shown in figure 1 (which is known as a continuity tester). As you can see, there is a break in the electrical path between the battery and the lamp. Using various household items (such as a plastic pen, an eraser, a ceramic plate, etc.) try to complete the circuit and see if the lamp lights. |
You will notice that metallic items cause the lamp to light while most others do not. Try using the graphite (also known as the ‘lead’) from a pencil. You will notice that this material allows the bulb to light although perhaps not as brightly, depending on how long or short the graphite is. This is because the graphite, although a conductor presents a certain amount of resistance to the flow of electricity. Using this device, we can characterize solid materials as either conductors or non-conductors. Non conductors are also known as insulators. There are many solid materials that have intermediate properties between conductors and insulators, like graphite.
|
What about water? Let’s modify the circuit we used earlier to now measure the conductivity of water (see figure 2). Instead of a light bulb to indicate the flow of electricity, now we will use an ammeter set to the microamp scale. This setup will be much more sensitive and will allow us to actually measure the electrical flow. Fill the container with distilled water and place the electrodes in the water as shown. Be careful not to touch the electrodes to each other. If there is any indication of current flow on the meter, it should be very small. This small current is due to the presence of H+ and OH– ions produced when a few of the water molecules dissociate and recompose spontaneously. The number of molecules doing this in any given moment is very small and this is why distilled water is a very poor conductor. Without disturbing the device, place a very small amount of salt in the water and with a plastic or glass utensil stir the water to mix the solution. As the salt crystals dissolve, the needle on the ammeter will move noticeably, indicating an increased conductivity of the water/salt solution. We used the same setup as before to help illustrate the similarity in the two devices, but you can also conduct the same experiment without the battery, but with an ohmmeter set to read resistance, as the ohmmeter has an internal battery and makes the setup less complicated. |
What happened? Why did the salt make the water more conductive to electricity? Kitchen salt consists of sodium and chlorine molecules (NaCl). The water not only separates the salt molecules one from another, but also separates the chlorine and sodium from each other. There is one change in the chlorine and sodium atoms as they separate. Elemental sodium weakly holds one excess electron and elemental chlorine has a strong attraction for a free electron, therefore as the two elements separate from each other the sodium gives up one electron to the chlorine. In this way, the sodium becomes electrically positive and the chlorine becomes negative. They have become ions. The molecules of salts, acids and bases become ions when dissolved in water. They dissociate into particles of opposite charges. It is the ions that render the water conductive to electricity. As a matter of fact, the positive ions migrate to the negative electrode and vice versa for the negative ions. In our experiment, the Na+ and Cl– ions made it possible for the distilled water to conduct electricity. This type of solution is called an electrolyte. Not only water, but other liquids as well can ionize various substances and result in electrolytic solutions.
If you add more salt to the solution you have been working with, you will see that the needle indicates increased current flow. The conductivity of a solution is proportional to the concentration of ions in the solution.
Let’s try the same experiment again, but in place of salt we will use an acid – vinegar for example. Refill the original container with fresh distilled water and stir in a few drops of vinegar while watching the ammeter. Try another time with a base such as household ammonia. Compare the conductivity of tap water to that of distilled water. You will find that the tap water is a good electrical conductor. This is why it is wise to be very careful around electricity when your hands are wet. Many people have been killed by appliances like hair dryers when used around water.
You should note that in contrast to the conduction of electricity in a solid, such as a metal wire, current that passes through an electrolyte moves ions to the electrodes where they undergo chemical reactions. In a wire this does not occur. Additionally, reactions in solution tend to polarize the electrodes. This phenomenon is what causes a battery to wear out with use and the measurements that we make with our experiments to change over time. In commercial conductivity meters, the electrodes are made of a non-reactive metal and use an alternating current at low voltage.
How does electricity flow in a metal if there are no ions moving around? In metals, free electrons carry the current. In a metal wire, all the atoms share the electrons in their outermost electron shell and these electrons are free to move anywhere within the wire. If a voltage is applied across a metallic object, electrons enter one side, exit at the other and within the object there is an electrical current.
The passage of electrons in a conductor causes a rise in the oscillations of the atoms in the crystal lattice. This corresponds to a rise in the temperature of the object (the Joule Effect). This phenomenon is used to produce light in an incandescent lamp. The current of electrons flowing through a lamp filament causes a temperature rise of thousands of degrees. At this high temperature the filament emits intense light.
Why don’t plastics, rubber and ceramics conduct electricity? This is due to the different molecular bond, called a covalent bond that binds these atoms to one another. In contrast to the metallic bond that we discussed in metals, a covalent bond does not allow the electrons to move freely within the body of the object. Electrons are exchanged only within the confines of each individual molecule and cannot move from molecule to molecule. Since there is no movement of electrons within the piece, and no movement of ions because the material is solid, there can be no flow of electrical charges through the material.
Despite what we have said about conductors and insulators, given a high enough voltage and a thin layer of the material in question, all materials will conduct electricity. Therefore, if we consider all materials to be conductors, it becomes necessary to know each material’s electrical conductivity.
In this experiment we have seen that various solids will conduct electricity better than others will (metals vs. rubber, plastic, ceramics). We have also seen that some liquids are better conductors than others are. While a solid’s conductivity is dependent upon the movement of electrons within the material’s crystalline structure, a liquid’s conductivity is dependent upon the movement of ions.
Materials: – a lemon |
|
Roll the lemon firmly with the palm of your hand on a tabletop or other hard surface in order to break up some of the small sacks of juice within the lemon. Insert the two metal strips deeply into the lemon, being careful that the strips not touch each other. Using the voltmeter, measure the voltage produced between the two strips (figure 3). It should show to be about one volt.
It would be nice to be able to illuminate a light bulb using your new lemon powered battery, but unfortunately it is not strong enough. If you were to try to light a bulb using this setup, the voltage across the strips would fall immediately to zero. Given this, if you want to demonstrate that the current produced by this battery is capable of powering something, try with a small device that uses an LCD display. A clock or a thermometer usually works well. An LCD display consumes an extremely small amount of current and your lemon battery is able to adequately drive this type of device. Remove any conventional battery that is in your clock or thermometer and power it with your lemon battery. You should see the device recommence functioning normally. If not, try swapping the polarity of the electricity from your lemon battery. This system allows you to demonstrate that the battery is producing energy even if you don’t have a voltmeter.
How does this battery work? The Copper (Cu) atoms attract electrons more than do the Zinc (Zn) atoms. If you place a piece of copper and a piece of zinc in contact with each other, many electrons will pass from the zinc to the copper. As they concentrate on the copper, the electrons repel each other. When the force of repulsion between electrons and the force of attraction of electrons to the copper become equalized, the flow of electrons stops. Unfortunately there is no way to take advantage of this behavior to produce electricity because the flow of charges stops almost immediately. On the other hand, if you bathe the two strips in a conductive solution, and connect them externally with a wire, the reactions between the electrodes and the solution furnish the circuit with charges continually. In this way, the process that produces the electrical energy continues and becomes useful.
As a conductive solution, you can use any electrolyte, whether it be an acid, base or salt solution. The lemon battery works well because the lemon juice is acidic. Try the same setup with other types of solutions. As you may know, other fruits and vegetables also contain juices rich in ions and are therefore good electrical conductors. You are not then, limited to using lemons in this type of battery, but can make batteries out of every type of fruit or vegetable that you wish.
Like any battery, this type of battery has a limited life. The electrodes undergo chemical reactions that block the flow of electricity. The electromotive force diminishes and the battery stops working. Usually, what happens is the production of hydrogen at the copper electrode and the zinc electrode acquires deposits of oxides that act as a barrier between the metal and the electrolyte. This is referred to as the electrodes being polarized. To achieve a longer life and higher voltages and current flows, it is necessary to use electrolytes better suited for the purpose. Commercial batteries, apart from their normal electrolyte, contain chemicals with an affinity for hydrogen which combine with the hydrogen before it can polarize the electrodes.
Materials: – a strip of copper |
|
Prepare a concentrated solution of copper sulfate in distilled water and another solution of similar concentration of zinc sulfate in distilled water. For both of these solutions, use about 10-30 grams of dry chemical per 100cc of distilled water. Construct a setup as shown in figures 4 and 5. Pour the CuSO4 solution in with the copper electrode and the ZnSO4 solution in with the zinc electrode. When you measure the voltage across these electrodes, you should find it to be about 1.1 volts. Compared to the Lemon Battery, the Daniell’s Cell puts out a higher power and lasts much longer. Even so, you would need electrodes with much greater surface area and more concentrated electrolyte to be able to power a very small light bulb with this device. Try instead a LED.
|
How does the Daniell’s Cell work? As we have said, the reactions at the electrodes furnish charges that allow the battery to produce electrical current for extended periods. In the Daniell’s Cell, the copper strip attracts electrons from the zinc strip. These electrons pass through the wires of our external circuit. As the copper electrode receives electrons, free positive ions in the solution arrive to equalize the charges. Positive copper ions (Cu++) are attracted to the charged copper electrode where they receive two electrons and become neutral and deposit on the electrode in metallic form. The positive zinc ions (Zn++) move to the porous vase. For each copper atom that is deposited on the copper electrode, a zinc atom goes into solution, giving up two electrons to the zinc electrode. |
The reactions at the electrodes can be represented by this formula:
Zn ==> Zn++ + 2e–
Cu++ + 2e– ==> Cu
These reactions result in the dissolution of zinc atoms in their ionic form, which corresponds to the deposition of copper ions in their metallic form:
Zn + Cu++ ==> Zn++ + Cu
The electrons made available by the zinc atoms pass through the lamp filament, produce light through the joule effect and eventually reach the copper electrode. These electrons account for the current that is produced by the battery and is used by the lamp. If we didn’t have the porous vase, the Cu++ ions would go directly to the zinc electrode and pick up free electrons, thereby bypassing the external circuit and stopping the current flow through the wires and lamp. The battery would no longer work. Because the copper electrode attracts electrons from the external circuit, it is considered the positive pole of the battery.
|
In a battery, there is always a flow of electrons in the external circuit (the electrical circuit or device) and a corresponding flow in the internal circuit (the electrolytic circuit). Like any battery, the Daniell Cell does not last forever, but only as long as there are Cu++ ions available and the zinc electrode is not consumed. In reality, the production of current diminishes as the concentration of the electrolyte bathing the zinc electrode increases and that bathing the copper electrode decreases. In fact, the positive ions produced by the zinc electrode need SO4 ions to balance the charges. The exact opposite occurs in the copper solution, which becomes scarce of positive ions. |
Since the electromotive force of a battery is dependant not only upon the nature it’s components, but also upon the concentration of it’s electrolytes, the gradient of concentrations that results from the production of electricity causes the battery to generate lower and lower voltages and currents until finally it is considered dead. At the end, Zn++ ions finally reach the copper electrode, surrounding it and blocking any further movement of Cu++ ions by polarizing the electrode.
You can also build a Daniell Cell without a porous vase by using what is known as a Saline Bridge (fig. 6 and 7). This device is made by using a “U” shaped tube filled with saline solution. You can make one with the plastic tube called out in the materials list earlier. Fill the tube with a Potassium Nitrate (KNO3) or Salt (NaCl) dissolved in distilled water (about 10 grams of chemical per 100 cc of water). Plug the ends with the cotton so that the saline solution will remain in the bridge and not mix with the electrolytes. The bridge serves the same purpose as the porous vase, acting as a barrier between the two different electrolytes while allowing the flow of charges. |
|
If you want higher voltages, you can connect multiple Daniell Cells in series as shown in figure 8. Note that between one cell and another, the connection is a metallic wire rather than a saline bridge.
|
Materials: – two strips of copper |
The setup of this experiment (fig. 9) is very similar to the Daniell Cell’s setup (fig. 4 and 5). This battery takes advantage of the tendency of two solutions having different concentrations to reach the same concentration level. In contrast to the Daniell’s Cell, we use only one material for the electrodes, in this case copper. As electrolyte, use copper sulfate in two concentrations, one using 30 grams of chemical in 100cc of water, the other using two grams of chemical in 100 cc of water. As you place the voltmeter in the circuit, it should indicate the voltage generated by the cell.
What is happening in this battery? The electrodes are undergoing a similar reduction reaction, but in two different concentrations. Due to this difference, there is a difference in potential (voltage) between the electrodes. At the anode, copper atoms dissolve into solution and give up electrons, while at the cathode, copper ions deposit on the electrode and acquire electrons. Cu ==> Cu++ + 2e– You can build a smaller, simpler version of this battery by substituting a “U” shaped tube in place of the porous vase. In the center of the length of tubing, tightly press a quantity of cotton in order to form a barrier between the different concentrations of solutions and prevent premature mixing. Add the solutions and the electrodes. In this model the battery will not last very long, just until the solutions equalize across the cotton barrier. |
|
Materials: – six strips or discs of copper |
|
In his famous experiment in the year 1800, Alessandro Volta used a solution of sulfuric acid as the electrolyte. In high concentrations, this acid can be very dangerous. If it gets on the skin or in the eyes, it can cause very serious burns and can permanently blind. Since we can build a functioning version with other types of electrolytes, in this experiment we will use a more benign substance, like those specified in the materials list – a solution of copper sulfate, for example. Despite this small modification in Volta’s Pile, our intended result – the production of electricity by chemical means, can be again demonstrated. If you decide to try the experiment using sulfuric acid, use a low solution of it and an adult must be present to avoid any dangers.
Place each zinc disc on a copper disc. You should have six sets of Cu-Zn disc pairs. As shown in fig. 11, build a stack or “pile” of these pairs of discs, with a disc of electrolyte soaked filter paper separating each pair from its neighbors. Be careful that the solution does not dribble down the side of the stack as this can cause a short circuit between the elements of the pile. Note that the sequence of the elements is as follows: Cu, Zn, electrolyte, Cu, Zn, electrolyte, etc. When the device is all arranged as specified, measure the voltage between the the bottom element of Cu and the top element of Zn. You should see 6.6 volts or 1.1 volt per pair of elements. The voltage generated is also dependent upon the electrolyte used and it’s concentration. As you may have done with the lemon battery, try to power a small LCD electronic device such as a clock, thermometer or even a calculator.
This invention in 1800 spurred intense research in the field of electricity. About a century later, this research will have culminated in electric lights, the telephone and radio receivers in millions of homes. Today, electricity is an important part in every moment of our lives.
MEASURING POTENTIALS OF REDUCTION
Another method of explaining the function of a battery lies in the oxidation reduction reactions at the electrodes. Oxidation reduction reactions involve the transfer of electrons from one chemical element to another. The tendency of an element to acquire or give up electrons is measured as electrical potential compared to that of a special hydrogen electrode, which is considered by convention to have zero electrical potential at 25°C. At this electrode, the following reaction occurs:
2H+ + 2e– <==> H2
To make these measurements, use is made of a cell with one electrode of hydrogen and the other of the material to be measured. The voltage produced indicates the potential of reduction of the new material in terms of positive or negative volts in respect to the hydrogen electrode. Chemical elements that have a positive potential of reduction tend to be reduced, that is to acquire electrons, while elements that have a negative potential tend to oxidize, that is to give up electrons.
At this point, the voltage produced by a battery can be calculated by finding the difference between the potentials of reduction of the two half cells: E = E1 – E2 as shown below.
reaction | potential of reduction (V) | |
---|---|---|
E1 | Cu++ + 2e– = Cu | + 0.342 |
E2 | Zn++ + 2e– = Zn | – 0.762 |
In this case, the voltage generated would be:
E = +0,342 – (-0.762)
E = +1.104 V.
You can make a battery from many different materials. It is possible to calculate in advance the voltage to expect from various materials by using the standard potential of reduction for the materials in question. This information can be found in any chemistry textbook.
Let’s get started with the experiment.
|
Materials: – a strip of copper |
Construct a setup such as that shown in fig. 12, very similar to the Daniell’s Cell with the saline bridge. Fill the container having the copper conductor with copper sulfate solution in the concentration of 1M. Fill the other container with the appropriate solution also in the concentration of 1M. Connect the negative side of the voltmeter to the copper electrode and the positive side to the electrode of test material and measure the voltage.
In order to get valid test measurements, it is necessary to have clean electrodes. Use the sandpaper to clean off any oxides or any other coatings or contamination so that the surfaces of the electrodes are clean and bright. Some metals oxidize very easily, such as aluminum, titanium, and magnesium. In the case that you are using a metal that oxidizes quickly, clean the electrode with sandpaper just before placing it in the electrolyte and then, during the experiment, wait a few moments for the chemical reaction to remove the slight amount of oxide that formed while you were cleaning it. You will see the voltage slowly rise to a maximum value. Note this reading as it represents the potential of reduction of the material under test with respect to copper. If the voltage starts immediately to drop, use the highest observed reading as the maximum value.
The voltages that you record are relative to copper. In order to obtain the value relative to hydrogen, that is the standard value, you would factor out the standard value of copper (Cu/Cu++). For example, if you measure the voltage between zinc and copper with this setup, you should see -1.1 volts output. By adding -1.1 and +.34 (the standard value for copper) you get -.76 volts which is exactly the potential of reduction for zinc ( Zn/Zn++) relative to hydrogen.
Compare the values you measure with your apparatus to the tables of standard potentials of reduction that you find in a chemistry text. You will probably find that the values you get using your setup will be close, but not exact matches to the standard values, and certainly close enough to make interesting observations about various materials.
Try many different materials – various metals, different alloys, and even conductive plastics, rubber, ceramics. Some researchers are working on batteries that have electrodes made of plastic. A battery made of plastics would have the advantage of being very light. Lightweight batteries would be necessary to realize lightweight electric vehicles.
|
Materials: – two copper strips |
Oxireduction reactions involve the exchange of electrons between one chemical and another, always moving toward lower energy levels. In an electrolytic cell, the chemical processes involved can be reversed simply by supplying electrical energy to the cell instead of drawing it from the cell. Now we will use this principle to deposit metallic copper on an electrode.
EXPERIMENT 1: CuSO4 in solution and copper electrodes.
Construct a setup such as that shown in figure 13. Use a solution of copper sulfate as the electrolyte (30g. of CuSO4 per 100 cc of water) and strips of copper as the electrodes. In this cell, the sulfate ions remain in solution, while copper ions are deposited on the cathode and dissolved from the anode. As the copper is constantly being replenished into solution from the anode, eventually it will be consumed entirely and redeposited atom by atom on the cathode. Any impurities present in the anode will settle out of solution at the bottom of the container. The copper deposited at the cathode is very pure. In fact, this method is used in industrial environments to purify metals, the resultant being called “electrolytically pure”. You can easily verify and monitor the progress of this transfer of metal from anode to cathode by weighing the electrodes before starting and at various times during the experiment. By changing the voltage across the cell, you can control the current flow. Make a chart of the rate of deposition on the cathode as compared to the current flow over fixed periods of time.
EXPERIMENT 2: CuSO4 solution and strips of zinc and iron.
|
Perhaps you are wondering why we haven’t been using a cathode of zinc which would make the deposition of copper easily visible. By virtue of it’s bold color, copper would be easily visible on zinc which is a dull gray color. This way, we wouldn’t need to use the scale to verify the deposition of copper. The problem is that the copper would begin depositing on the zinc before the current is even turned on. Copper ions from the copper sulfate would spontaneously deposit on the zinc strip. As you recall from earlier experiments, the copper atoms have a much stronger attraction for electrons than the zinc atoms. As the zinc strip is submerged in the solution containing copper ions, some zinc atoms are dissolved in solution as Zn++ ions and the same number of Cu++ ions are deposited on the zinc where they pick up the electrons lost by the zinc atoms and become electrically neutral. The zinc strip gets covered in a visible layer of copper. Normally zinc gets a deposit that appears dark in color, is of a powdery texture and has a dendritic, crystalline form. If you use an iron strip instead of zinc, you get a much more compact, metallic deposit of copper (fig. 14). Experiment freely with this phenomenon. |
Experiment 3: CuSO4 solution and cathode of stainless steel.
Stainless steel is well adapted for use as a demonstrator of the deposition of copper on an electrode. It has a gray color that makes copper deposits easy to see and it will not spontaneously pick up copper deposits from copper sulfate solution. It is necessary to run a current through the solution in order to drive the copper deposits onto the stainless steel electrode. This is due to the fact that the stainless steel has a potential of reduction greater than that of copper. As shown in figure 13, use your strip of stainless steel as the cathode and apply electric current to the circuit. You will see that the portion of the cathode submerged in the electrolyte acquires a light coating of copper, as shown by the change in color.
In the course of these experiments, you can verify Faraday’s law, which states that the quantity of electrons furnished is equal to the ionic charge transferred. The weight of the materials transformed is directly proportional to the amount of current supplied and the molecular weight of the ions and inversely proportional to the number of charges the material possesses in the ionic state. By way of example: a certain quantity of current will deposit a certain number of ions with a charge of 1, and half as many ions that have a charge of 2.
Electrolytic deposition is widely used in industry as a surface treatment on metals. These treatments can protect the underlying structure from oxidation, or can serve a decorative function. Jewelry makers deposit very thin layers of gold on base metals to achieve a product which is far less costly, yet has all the appearance of solid gold. In electronics, gold is widely used to coat electrical contacts and is valued for its resistance to oxidation and it’s long-term stability. Galvanic techniques can also be used for other surface treatments of metals for decorative and protective purposes. Aluminum is often “anodized” which means that it is used as the anode in a process that oxidizes the surface, protecting it from corrosion and achieving various colorations all the way to black.
In the course of these experiments, you have become familiar with various concepts such as the conductivity of solutions by way of dissolved ions. you have seen redox reactions at work, and that they can be reversed by running current through the circuit. You have learned to measure potentials of reduction of various materials and to predict the voltage generated by a battery when you know it’s material components. You can build a working battery from various materials, including lemons, tomatoes, potatoes, almost any fruit or vegetable. Up until now, batteries may have seemed mysterious, but now we are among the few that have a working knowledge of how and why they work. You can amaze your friends by building a battery out of almost anything, but more important than this, now the study and mastery of this subject will be easier and more meaningful. |
|
منبع:http://www.funsci.com/fun3_en/electro/electro.htm